How The Plan For Releasing Treated Water In Fukushima Works
Technological causes, expected environmental impacts, foreign policy implications and background – A personal expert analysis
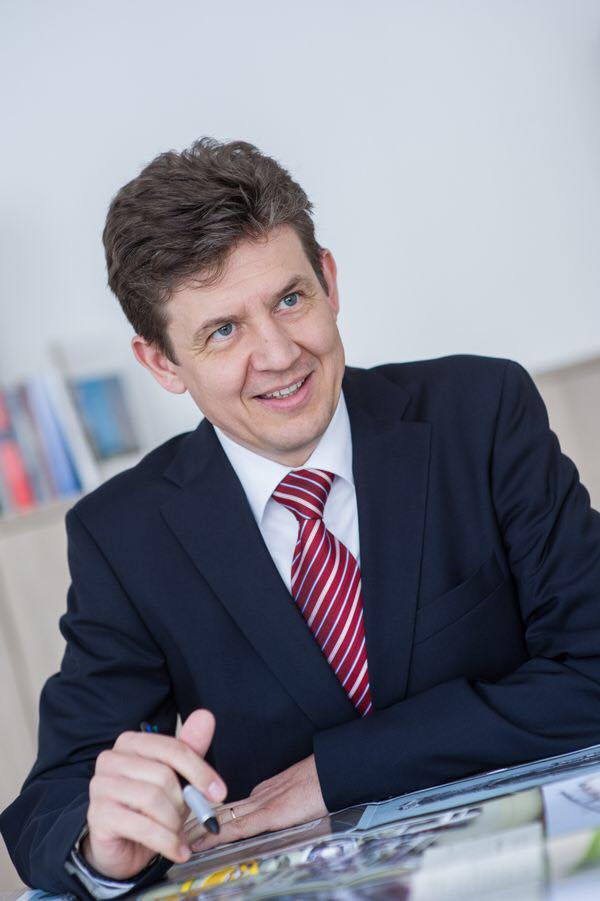
Prof. Dr. Attila Aszódi
In recent days, the international press has focused on the Fukushima nuclear power plant’s wastewater treatment plans and the release of tritium-contaminated water into the ocean. Rafael Mariano Grossi, Director General of the International Atomic Energy Agency (IAEA), visited Japan and several countries in the Pacific region (including South Korea and New Zealand), and the IAEA issued a new technical report, now in its 5th edition, with the title “IAEA Review of Safety-Related Aspects of Handling ALPS-Treated Water at TEPCO’s Fukushima Daiichi Nuclear Power Station, Report 5: Review Mission to NRA (January 2023)“. As the title of the report indicates, the IAEA review mission did not visit the Japanese Nuclear Safety Authority now, but back in January 2023. What has gotten attention now is that the final version of the IAEA report has just been issued, and the IAEA Director General has travelled to the region to present this. (ALPS stands here for Advanced Liquid Processing System, details see below.)
The work of the IAEA is very much needed (also) here because there are many questions both in Japan and in the region (and indeed the world) about the waste management plan that the Japanese government presented back in early 2021. As the plan involves the concept of discharging a very large amount (1.3 million m3) of radioactively contaminated water into the sea, spread over a 30-year period, it has been the subject of much criticism over the past 2 years (see later for the technology).
It is important to clarify at this point that the IAEA is not a licensing authority and cannot approve or reject these plans. Nuclear safety and environmental safety are national competencies everywhere, in this case, the Nuclear Regulation Authority (NRA) of Japan should approve the plans and subsequently verify the proper implementation of the approved plans. The IAEA cannot and does not take over any powers from the Japanese authority. The role of the IAEA is to compare the licensing process and the technical and administrative solutions to be licensed against IAEA requirements and international best practices, to provide independent review and to ensure a high degree of transparency by making the results available to the international professional and non-professional public and to governments.
Why and from where is there contaminated water at the Fukushima-Daiichi nuclear power plant?
On 11 March 2011, a massive magnitude 9 earthquake struck the east coast of Japan. The earthquake damaged many buildings, roads, bridges and railways, and disrupted the power grid in the northern part of the country. Just as Japanese high-speed trains automatically stopped on the open railway tracks at the time of the earthquake, nuclear power plants in the affected areas also automatically shut down, but a massive number of electricity grid poles crashed, causing the power grid to collapse and temporarily cutting off electricity supply to many areas. This is what happened also at the Fukushima-Daiichi (Fukushima I) nuclear power plant. Although the earthquake caused damage, the plant was cooled down normally and, in the absence of external electricity, the emergency diesel generators performed their important function.
However, about 45 minutes after the earthquake, a huge tsunami hit the affected coastline. The Fukushima nuclear power plant was not prepared for the 30-metre-high tsunami waves. The tsunami destroyed the water intake facilities vital for cooling the plant (a Loss of Ultimate Heat Sink (LUHS) accident – i.e. ocean water could not be used to cool the plant), mechanically damaged the diesel generators and submerged the backup batteries located in the buildings (so-called SBO, Station Black-Out event), leaving the plant not only without cooling water but also without emergency power (a combination of LUHS and SBO, a highly complex accident situation for which nuclear power plants were not typically designed before 2011). Therefore, the reactors could not be cooled, fuel and then large-scale core damage occurred in reactors No. 1, 2 and 3, and the explosion of hydrogen gas from fuel overheating caused significant damage to the structure of the buildings and their technological systems. An unplanned situation was created in which the cooling of the reactors in the planned, well-established way was not possible anymore, and normal cooling could not be restored.
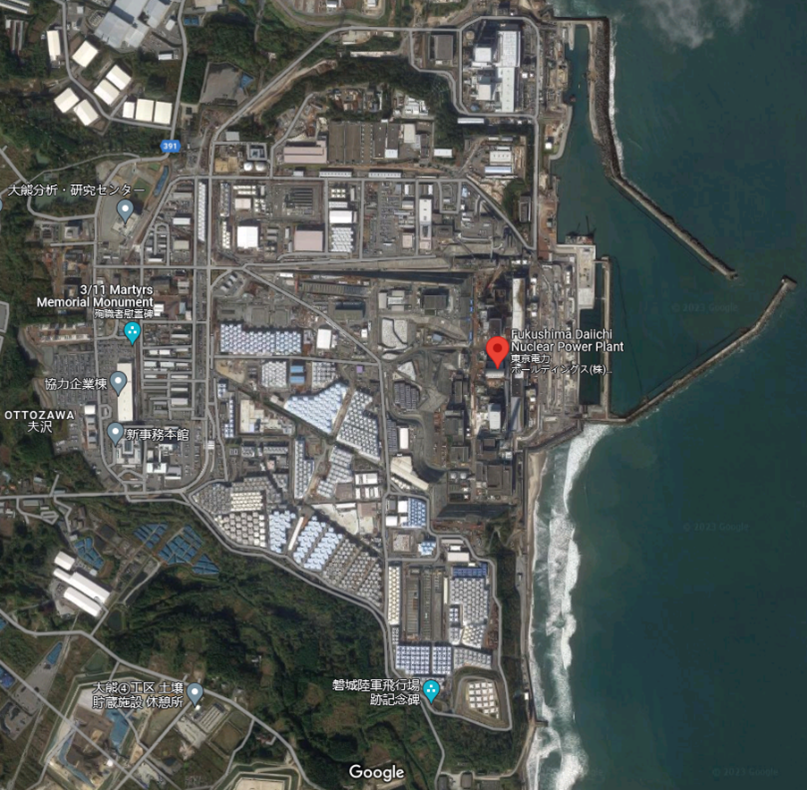
Figure 1: Google Maps satellite image of the Fukushima I nuclear power plant site, showing the huge mass of wastewater storage tanks in the middle and bottom half of the image (source: Google Maps)
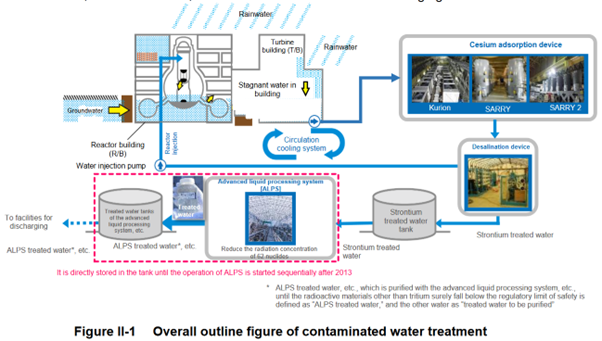
Figure 2: Principle flow diagram for the generation and treatment of contaminated water (source: TEPCO [5])
This has led to a situation where the site has had to be stacked with large tanks, each with a storage capacity of around 1300 m3. In total, there are 1000 such large capacity tanks on the site (see Figure 1), in which this water is stored, filtered and treated, but the water left over after filtration also has to be collected and stored. In total, there is currently 1.33 million m3 of contaminated water in tanks, with a total storage capacity of 1.37 million m3 for the entire tank farm, which is slowly running out of storage capacity.
If no action is taken, by 2024 the tank farm would be full and there would be nowhere to put the wastewater pumped out from the reactor buildings, and the large number of tanks and the water stored in them will also hinder the planned decommissioning of the power plant. The current situation is also unsustainable because of the risk of natural hazards in the area (e.g. an extreme monsoon or another major earthquake or tsunami), which could damage the tanks and release their contents uncontrolled into the sea.
TEPCO, the operator of the plant, investigated several options, and the solution described below was the best, so they asked the Japanese government for permission, which was granted. (As another option, the Japanese authorities have investigated the possibility of evaporating the water contaminated with tritium. The calculations showed that this solution would require much more energy and would also result in a higher dose of the population, as the water content of the vapour would later be precipitated as rain and could affect many more inhabited areas.)
Let’s review the technology currently in use!
The wastewater is pumped out of the buildings, from where it is pumped to a tank and temporarily stored. The two main contaminant substances, caesium-137 and strontium-90 isotopes, are then removed. These are present in large quantities in the water, are highly active and emit high-energy radiation that is harmful to human cells, so it was important to filter them out from the very beginning.
The wastewater is then fed into the ALPS technology, which removes the remaining 60 isotopes from the water after the previous purification step. This means that virtually all isotopes are removed from the water, except for tritium (hydrogen-3) and carbon-14. The licensee (TEPCO) is required not only to reduce the activity concentration of each isotope in the discharged water below the limit for that isotope (see limits in the table in Figure 3) but also to comply with a combined activity concentration limit for the 62 isotopes (in the form of a ratio not described here in details). This should ensure that the ocean, as a natural biological medium receiving the contaminated water, is subjected to limited exposure and that no significant increase in the concentration of radioactive substances in living organisms is expected as a result of the release.
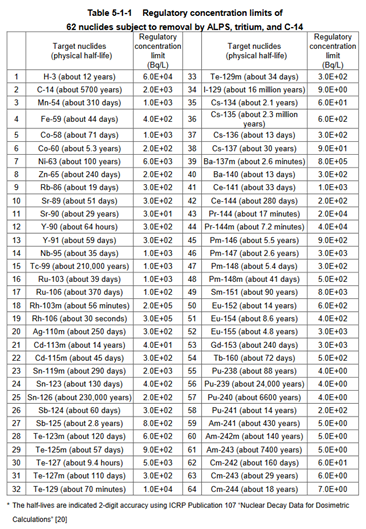
Figure 3: Official limits for the 62 isotopes extracted by the purification system, plus tritium and C-14 (Source: TEPCO [5]). Bq/litre is a unit of activity concentration; it expresses how many radioactive decays occur in the given amount of material. 1500 Bq/litre means here 1500 radioactive decays per second in 1 litre of water.
What is tritium, what is its significance and why can’t it be removed from water?
Tritium is a heavy isotope of hydrogen. While the most common light hydrogen has only one proton in its nucleus, tritium has two neutrons in addition to the proton, giving it an atomic number of 1 and a mass number of 3.
Tritium is a naturally occurring element, formed by the nuclear reaction of cosmic ray particles with nitrogen in the high atmosphere. It has a half-life of 12.3 years, so its production from cosmic rays and depletion from radioactive decay creates an equilibrium concentration of tritium in nature. Its atmospheric concentration has been significantly increased by experimental nuclear bomb explosions.
From a radiation protection point of view, tritium is one of the least dangerous radioactive isotopes: it is a low-energy beta emitter. The beta particles emitted during the decay of tritium are absorbed in the air as thin as 5-6 mm thick layers of air or even in a sheet of paper. It is already trapped on the outer layer of the skin, so its dosimetric significance as an external radiation source is negligible.
However, if tritium is ingested with food or drink, it can enter the body where the skin’s protective effect is no longer present. At the same time, its low-energy beta radiation means that it has very little cell-destroying capacity. An important property is that tritium does not accumulate in living organisms. While iodine, for example, is collected and accumulated by the thyroid gland, tritium is neither accumulated by the human body nor by its organs. The biological half-life of tritium in the human body is one to two weeks (compared to a physical half-life of 12.3 years), which means that the tritium ingested is rapidly excreted by the body, mostly in the urine. This significantly reduces the dose that can be absorbed from tritium. Therefore, in many countries, the permissible tritium activity concentration in drinking water is 1500 Bq/litre, but there are places where the limit is 10,000 Bq/litre.
Tritium is constantly being produced in nuclear reactors. In pressurised water reactors, mainly from boron used for neutron absorption or from lithium used for water treatment, in a neutron absorption-induced nuclear reaction. More tritium is produced in heavy water-moderated reactors, so-called CANDU reactors, moderated by water containing the other heavy isotope of hydrogen, deuterium (mass number two). In these reactors, tritium is mainly produced from deuterium by neutron capture. The processing and reprocessing of spent fuel is also a major source of tritium.
The difficulty with treating tritium is that this element is found in the same water molecule as H2O, and it is very complicated to filter out tritiated water molecules from water. This is not impossible, in the case of the CANDU reactors mentioned above they can process and remove tritium from about 2000 tonnes of cooling water per year, but in the case of Fukushima, we are dealing with a much more diluted tritiated water mixture. Removing tritium from this 1.3 million m3 of water would be too expensive and would take too much time.
The planned process of discharge
The water treated by ALPS is not completely uniform, its composition depends on the time of origin, the time of treatment, and the properties of the ALPS technology at the time (which has also evolved over time). For this reason, any water mass that is prepared for discharge will undergo further ALPS purification (secondary ALPS treatment) before discharge. To carry out this discharge process, TEPCO will set aside a separate group of tanks, consisting of 3×10 tanks. The operator will rotate between them to carry out the functions to be performed, as illustrated in Figures 4 and 5:
- Receiving water from ALPS technology
- Storage of water while awaiting the results of its analysis and confirmation of its composition
- Storage of water that is ready for release (obviously the release will be slow, continuous, and diluted, therefore the 3rd group of tanks is needed)
The tank farm is located on the site, above sea level. The water for discharge will be pumped down to sea level, where it will be mixed with seawater taken from the Unit 5 intake shaft, which will then be pumped out through a subsea pipeline via a pipe installed on the seabed, 1 km from the coast. The discharge site is already in an area closed to fishing.
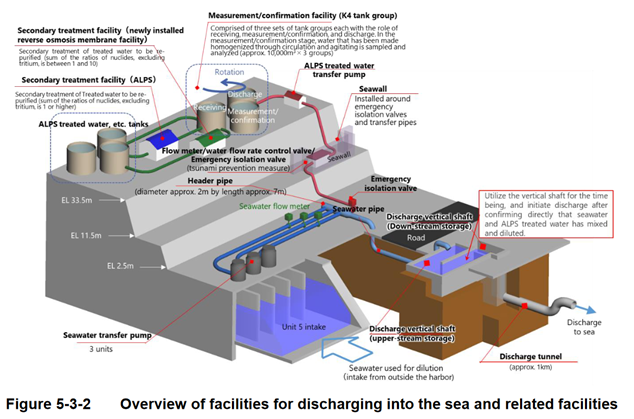
Figure 4: Systems used to discharge contaminated water (source: TEPCO [5])
The emission limit for tritium during operations will be 22 TBq per year, which is the same as it was the normal annual tritium emission from the Fukushima nuclear power plant before the accident. (Nuclear power plants emit small amounts of gaseous and liquid radioactive material during normal operation due to their technology, but this is strictly regulated and measured – the emission limits are chosen to avoid adverse effects on public health with a high level of safety.) So, despite the clean-up of the accident situation, there is no intention to apply an extraordinary, emergency discharge limit, and the release of water treated with ALPS technology will be within normal operational limits. In addition to the total annual discharge limit for tritium, a limit will also be applied to the discharge activity concentration, which is a concentration of 1,500 Bq/litre of tritium in the discharged water. This is the limit for the tritium activity concentration in drinking water in many countries. This limit for drinking water will be the same concentration that will be discharged to the sea at a distance of 1 km from the coast. In the mixing/dilution shaft shown in the bottom right-hand corner of Figure 4, the aim is to achieve a dilution of about 100 times during mixing to maintain the tritium activity concentration at the point of discharge at 1500 Bq/litre. The need for dilution also means that the tritium activity concentration in the wastewater stored in the tanks is much higher than the 1500 Bq/litre limit mentioned here.
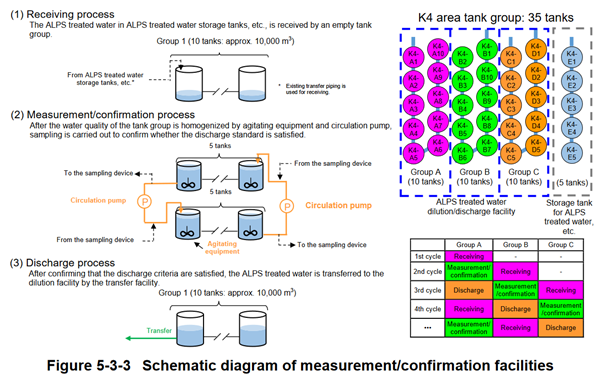
Figure 5: The main elements of the pre-discharge measurement and control system and the logic of their cyclic operation (source: TEPCO [5])
The Japanese nuclear safety authority, the NRA, has calculated the discharge limit on the basis that the population most exposed to radiation should not receive annual doses exceeding 50 µSv from these operations. This is the so-called dose constraint, which is only 1/20th of the annual dose limit for individuals in the Japanese population.
The most important species in the affected section of coastline in terms of discharges and the human food chain are seaweeds and halibut. To study them, the Japanese authorities have launched a major experimental programme. These marine organisms are kept in aquariums containing water treated with ALPS technology, observed and studied, and their isotope uptake is measured regularly, so that the isotope exposure of the local population through the food chain can be accurately measured. The licensee TEPCO has also submitted a detailed monitoring programme to the Japanese nuclear safety authority. Given that the release of the wastewater and the decommissioning of the huge tank farm is now expected to take three decades, a long-term investigation and monitoring programme is being established. According to the IAEA, the environmental monitoring and investigation programme is state-of-the-art, sufficiently detailed and capable of accurately collect the necessary data and monitor the environmental impact of the Fukushima discharges.
Protests against the plan and their international aspects
South Korea seems to be the most outspoken in protests against the discharge of water from Fukushima, along with Japanese fishermen, but many other countries in the Pacific region have protested against the plan. Fishermen’s protests are understandable: they fear that their catches will not be marketable. The transparent and continuous operation of the above-mentioned environmental monitoring programme could provide a reassuring answer to these concerns in time.
It is interesting that there are very intense protests from South Korea, when in fact the normal operational tritium discharge from South Korean nuclear power plants is almost ten times the planned annual discharge from Fukushima. In my view, the reason for these protests is, surprisingly, of a South Korean internal political nature.
South Korea is a presidential republic. Moon Jae-in was President of South Korea from 2017 to 2022, and as a liberal politician, he promoted a fundamentally anti-nuclear energy policy. In 2022, the conservative Yoon Suk Yeol won the presidency of the Republic of South Korea and made the development of nuclear power and renewables a cornerstone of his energy policy. So, there is a fundamental difference of opinion between the two major parliamentary parties in South Korea on nuclear energy, and I think this is reflected in the way how the country views Japan’s plans in relation to Fukushima.
It is worth noting that South Korea is at a huge distance from the Fukushima nuclear power plant: the east coast of South Korea, west of Japan, is more than 1,000 km from the damaged Japanese power plant even in a straight line, but if we consider that the Fukushima site is on the east coast of Japan, the water would have to travel some 1,800 km at sea to reach the coast of South Korea. Obviously, there is a huge dilution over this distance, so there is in fact no environmental risk to South Korea from the Japanese release plans.
Overall, I believe, in line with the IAEA report, that the releases from Fukushima do not pose an environmental threat to Japan or to countries in the region. The important and environmentally hazardous isotopes will be filtered out of the water and tritium will be released in compliance with the strict discharge limit.
Obviously, we are not happy about this release, as the waste management method most often used in the nuclear industry is to collect, concentrate and isolate the waste from the environment, but in this extreme situation, there is really no other viable solution left for Japan other than to filter out the isotopes that can be treated and release water contaminated with tritium that cannot be removed.
In my view, the key tasks are:
- to consistently implement the developed environmental monitoring programme,
- to communicate the results transparently and continuously, and
- to ensure that wastewater discharges are free from incidents and disruptions, not only now but throughout the planned 30-year implementation of the programme.
References:
- IAEA comprehensive report on the safety review of the alps-treated water at the Fukushima Daiichi nuclear power station, IAEA, 2023
- IAEA Review of Safety Related Aspects of Handling ALPS-Treated Water at TEPCO’s Fukushima Daiichi Nuclear Power Station, Report 5: Review Mission to NRA (January 2023)
- Fukushima Daiichi Progress in nuclear safety since 2011, IAEA, 2021
- Basic Policy on handling of ALPS treated water at the Tokyo Electric Power Company Holdings’ Fukushima Daiichi Nuclear Power Station, TEPCO, 2021
- Partial Revision of the Application for approval to amend the Implementation Plan for Fukushima Daiichi Nuclear Power Station as Specified Nuclear Facility; TEPCO partially revise Application for approval to amend the Implementation Plan for Fukushima Daiichi Nuclear Power Station as Specified Nuclear Facility, which submitted on Nov. 14, 2022 (Application No. FDEC-R4-143), as per the attached document, Tokyo Electric Power Company Holdings Inc., Application No. FDEC-R4-179, February 14, 2023
- List of presidents of South Korea, https://en.wikipedia.org/wiki/List_of_presidents_of_South_Korea
- Fukushima Daiichi und das Wasser, GRS, 2023.07.04., https://www.grs.de/de/aktuelles/fukushima-daiichi-und-das-wasser
Prof. Dr. Attila Aszódi
Full professor at the Institute of Nuclear Techniques of Budapest University of Technology and Economics. Currently, he is the dean of the Faculty of Natural Sciences at the Budapest University of Technology and Economics (Hungary). He took part in several projects of the International Atomic Energy Agency and the European Commission. He has more than 300 published scientific articles. His research interest is reactor safety, thermal hydraulics of nuclear reactors, Computational Fluid Dynamics, Particle Image Velocimetry, nuclear power plants, energy policy and sustainability.