Nuclear energy is a key component of the energy mix around the world. There are more than 400 nuclear power plants in operation, seventy under construction and several dozen new projects are under study.
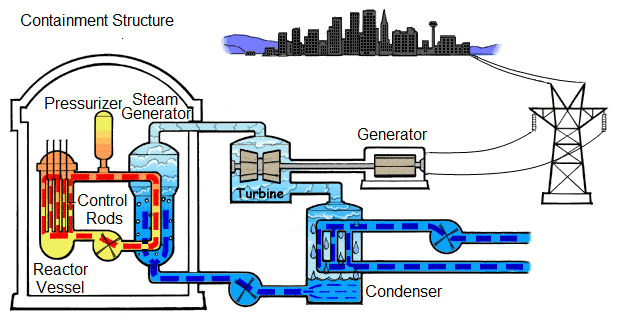
Figure 1: The different components of a Pressurised Water Reactor (PWR) power plant. (Source: US NRC)
A nuclear power plant can be compared to a steam engine. But, unlike a steam engine, the heating of the water is done by means of fissile material, and so without combustion.
In all nuclear power plants, electricity production comes from the fission of uranium or plutonium nuclei. When a nucleus of fissile uranium or plutonium is hit by a neutron, it breaks up. This nuclear fission produces more neutrons (two or three per fission) and creates new atoms (fission products such as xenon, krypton, iodine, cesium, …). This chain reaction releases large amounts of heat that can heat water to turn it into steam. This then rotates a turbine which is coupled with an alternator that produces electricity.
Differentiating nuclear reactors according to their design features is especially pertinent when referring to nuclear power reactors (see Types of Nuclear Power Reactors).
Nuclear power reactors can be classified according to the type of fuel they use to generate heat.
The only natural element currently used for nuclear fission in reactors is uranium. Natural uranium is a highly energetic substance: one kilogram of it can generate as much energy as 10 tonnes of oil. Naturally occurring uranium comprises, almost entirely, two isotopes: U238 (99.283%) and U235 (0.711%). The former is not fissionable while the latter can be fissioned by thermal (i.e. slow) neutrons. As the neutrons emitted in a fission reaction are fast, reactors using U235 as fuel must have a means of slowing down these neutrons before they escape from the fuel. This function is performed by what is called a moderator, which, in the case of certain reactors (see table of Reactor Types below) simultaneously acts as a coolant. It is common practice to classify power reactors according to the nature of the coolant and the moderator plus, as the need may arise, other design characteristics.
Reactor Type | Coolant | Moderator | Fuel | Comment |
Pressurised water reactors (PWR, VVER) | Light water | Light water | Enriched uranium | Steam generated in secondary loop |
Boiling water reactors (BWR) | Light water | Light water | Enriched uranium | Steam from boiling water fed to turbine |
Pressurised heavy water reactor (PHWR) | Heavy water | Heavy water | Natural uranium | |
Gas-cooled reactors (Magnox, AGR, UNGG) | CO2 | Graphite | Natural or enriched uranium | |
Light water graphite reactors (RBMK) | Pressurised boiling water | Graphite | Enriched uranium | Soviet design |
PWRs and BWRs are the most commonly operated reactors in Organisation for Economic Cooperation and Development (OECD) countries. VVERs, designed in the former Soviet Union, are based on the same principles as PWRs. They use “light water”, i.e. regular water (H2O) as opposed to “heavy water” (deuterium oxide D2O). Moderation provided by light water is not sufficiently effective to permit the use of natural uranium. The fuel must be slightly enriched in U235 to make up for the losses of neutrons occurring during the chain reaction. On the other hand, heavy water is such an effective moderator that the chain reaction can be sustained without having to enrich the uranium. This combination of natural uranium and heavy water is used in PHWRs, which are found in a number of countries, including Canada, Korea, Romania and India.
Graphite-moderated, gas-cooled reactors, formerly operated in France and still operated in Great Britain, are not built any more in spite of some advantages.
RBMK-reactors (pressure-tube boiling-water reactors), which are cooled with light water and moderated with graphite, are now less commonly operated in some former Soviet Union bloc countries. Following the Chernobyl accident (26 April 1986) the construction of this reactor type ceased. The operating period of those units still in operation will be shortened.
Plutonium (Pu) is an artificial element produced in uranium-fuelled reactors as a by-product of the chain reaction. It is one hundred times more energetic than natural uranium; one gram of Pu can generate as much energy as one tonne of oil. As it needs fast neutrons in order to fission, moderating materials must be avoided to sustain the chain reaction in the best conditions. The current Plutonium-fuelled reactors, also called “fast” reactors, use liquid sodium which displays excellent thermal properties without adversely affecting the chain reaction. These types of reactors are in operation in France, Japan and the Commonwealth of Independent States (CIS).
The Light Water Reactors category comprises pressurised water reactors (PWR, VVER) and boiling water reactors (BWR). Both of these use light water and hence enriched uranium. The light water they use combines the functions of moderator and coolant. This water flows through the reactor core, a zone containing a large array of fuel rods where it picks up the heat generated by the fission of the U235 present in the fuel rods. After the coolant has transferred the heat it has collected to a steam turbine, it is sent back to the reactor core, thus flowing in a loop, also called a primary circuit.
In order to transfer high-quality thermal energy to the turbine, it is necessary to reach temperatures of about 300 °C. It is the pressure at which the coolant flows through the reactor core that makes the distinction between PWRs and BWRs.
In PWRs, the pressure imparted to the coolant is sufficiently high to prevent it from boiling. The heat drawn from the fuel is transferred to the water of a secondary circuit through heat exchangers. The water of the secondary circuit is transformed into steam, which is fed into a turbine.
In BWRs, the pressure imparted to the coolant is sufficiently lower than in a PWR to allow it to boil. It is the steam resulting from this process that is fed into the turbine.This basic difference between pressurised and boiling water dictates many of the design characteristics of the two types of light water reactors, as will be explained below.
Despite their differing designs, it must be noted that the two reactor types provide an equivalent level of safety.
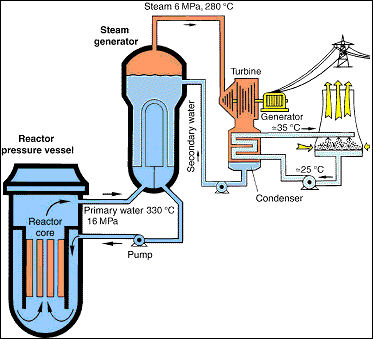
Figure 2: Schematic of a pressurised water reactor.
The fission zone (fuel elements) is contained in a reactor pressure vessel under a pressure of 150 to 160 bar (15 to 16 MPa). The primary circuit connects the reactor pressure vessel to heat exchangers. The secondary side of these heat exchangers is at a pressure of about 60 bar (6 MPa) – low enough to allow the secondary water to boil. The heat exchangers are, therefore, actually steam generators. Via the secondary circuit, the steam is routed to a turbine driving an alternator. The steam coming out of the turbine is converted back into water by a condenser after having delivered a large amount of its energy to the turbine. It then returns to the steam generator. As the water driving the turbine (secondary circuit) is physically separated from the water used as reactor coolant (primary circuit), the turbine-alternator set can be housed in a turbine hall outside the reactor building.
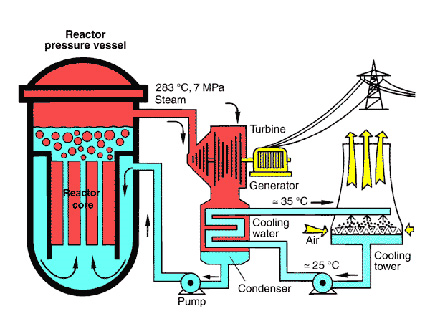
Figure 3: Schematic of a boiling water reactor. (From Informationskreis Kernenergie)
The fission zone is contained in a reactor pressure vessel, at a pressure of about 70 bar (7 MPa). At the temperature reached (290 °C approximately), the water starts boiling and the resulting steam is produced directly in the reactor pressure vessel. After the separation of steam and water in the upper part of the reactor pressure vessel, the steam is routed directly to a turbine driving an alternator.
The steam coming out of the turbine is converted back into water by a condenser after having delivered a large amount of its energy to the turbine. It is then fed back into the primary cooling circuit where it absorbs new heat in the fission zone.
Since the steam produced in the fission zone is slightly radioactive, mainly due to short-lived activation products, the turbine is housed in the same reinforced building as the reactor.
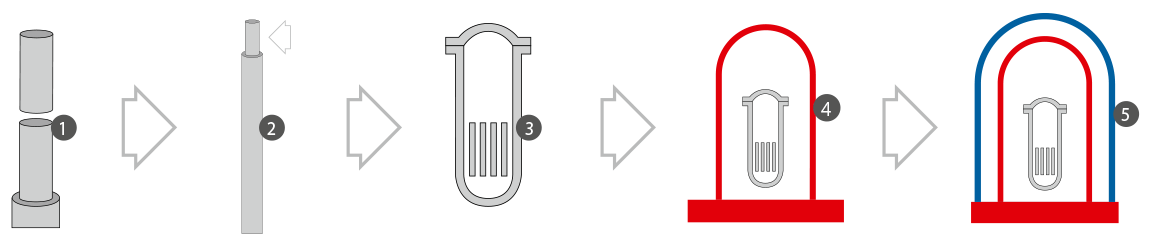
Figure 4: A series of five successive containment barriers completely isolate uranium and highly radioactive fission products. (Source: Forum Nucleaire Belge)
A series of five successive containment barriers completely isolate uranium and highly radioactive fission products. Three strong and waterproof physical barriers ensure the containment of radioactive materials. This triple layer protects against radiation and isolates radioactivity inside installations in all circumstances.
Barrier 1 and 2: pellets and fuel rods The uranium is converted into combustible ceramic pellets (1) which are introduced into a zircaloy protective metal sheath to form perfectly sealed fuel rods. The assembly of these rods will be the heart of the reactor. The pellets are in turn stacked in hermetic fuel rods (2).
Barrier 3: the reactor vessel These bars are then assembled into fuel elements and placed in the reactor vessel (3), whose steel wall is 25 cm thick.
Barriers 4 and 5: the reactor building A first enclosure prevents any release of radioactivity out of the reactor building (4); it resists strong pressure from the inside. A second reinforced concrete enclosure (5) protects the installations from external accidents. It is designed to deal with different scenarios of incidents or accidents, a fire, a flood, an earthquake, the impact of an airplane. A vacuum between the two enclosures prevents uncontrolled release of radioactivity to the outside.