The applications of nuclear are continually being furthered by innovative research.
In order to provide a reliable baseload supply to the energy grid which is also a clean, low carbon source of energy, nuclear power is required.
Three generations of nuclear reactors have provided clean electricity for over 50 years, however the next generation of reactors in development have more stringent requirements to meet; namely to minimize waste production and environmental impact, as well as increasing safety and proliferation resistance.
Fourth generation reactors use fuels more efficiently, which leads to a higher output with far less radioactive waste. Compared to previous generations; the new reactors can generate 50 times more energy with the same amount of uranium. These innovative reactors can even produce energy from existing radioactive waste1.
Currently the laboratories and research institutes are conducting tests on many different types of materials and fuels that might be suitable for these future nuclear reactors. More
For the development of the next generation of reactors, the Generation IV forum was set up. It carries out the research and development to establish feasibility and performance capabilities of the next generation nuclear fission energy systems. 100 experts evaluated 130 reactor concepts, and the forum selected 6 for further research and development. These are the gas-cooled fast reactor, lead-cooled fast reactor, molten salt reactor, supercritical water-cooled reactor, sodium-cooled reactor and the very high temperature reactor (Table 1).
Reactor Type | Description |
Gas-cooled Fast Reactor (GFR) | A high-temperature, helium-cooled fast spectrum reactor with a closed fuel cycle. |
Lead-cooled Fast Reactor (LFR) | A high temperature, fast spectrum reactor which is cooled by molten lead or lead-bismuth eutectic. There are a number of LFR concepts in development in Russia, China, the USA, Sweden, Korea and Japan |
Molten Salt Reactor (MSR) | The core of this reactor has the fuel dissolved in molten fluoride salt. |
Supercritical Water-cooled Reactor (SCWR) | High temperature, high-pressure, light-water cooled reactors which operate above the thermodynamic critical point of water (374°C, 22.1MPa) |
Sodium-cooled Fast Reactor (SFR) | Liquid sodium is the reactor coolant , and this reactor operates a closed fuel cycle. |
Very High Temperature Reactor (VHTR) | This reactor is primarily dedicated to the cogeneration of electricity and hydrogen, with the latter being extracted from water by using thermo-chemical, electro-chemical or hybrid processes. |
Cogeneration is the integration of nuclear power plants with other systems and applications (Figure 1). The heat generated by nuclear power plants can be utilized in a range of processes such as seawater desalination and hydrogen generation.
Depending on the type of commercial nuclear power reactor, they are best suited for different applications for cogeneration due to their operating temperatures (Table 2). Cogeneration will allow for reduction in the environmental impact in nuclear power and ensure more efficient use of generated heat. It will also allow for local district heating to be supported by lower temperature reactors, which will reduce the infrastructure needed.
Table 2 Different reactor types and potential cogeneration applications
Reactor Type | Operating Temperature | Cogeneration Application |
Light Water Reactor (LWR)
Heavy Water Reactor (HWR) Small Modular Reactor (SMR) |
280-325°C | District heating
Desalination |
High Temperature Gas Reactor (HTGR) | 750-950°C | Generation of process heat and hydrogen |
Supercritical Water Reactor (SCWR)
Gas Fast Reactor (GFR) Molten Salt Reactor (MSR) |
430-625°C
~850°C 750-1000°C |
Hydrogen production, process heat and desalination |
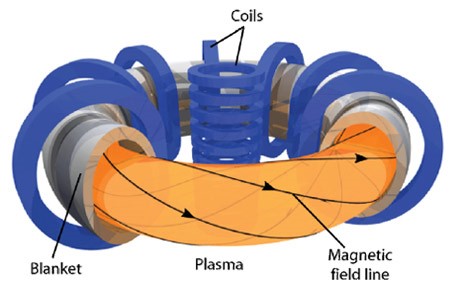
Figure 2 Tokomak plasma chamber. Image courtesy of EFDA.
A potentially limitless source of energy is nuclear fusion, a common process which generates energy through the fusion of light nuclei- hydrogen atoms. Through the collision of these nuclei, they fuse into heavier atoms and release enormous amounts of energy in the process. Fusion is the process which powers the sun and other stars in our universe2.
There has been a vast international effort to harness this process to produce energy on Earth. But in order to do so, gases need to be heated to extremely high temperatures of about 150 million °C- at which point it becomes a plasma. To reach such high temperatures, there must be powerful heating and little to no thermal losses. One way this is achieved is by using a tokamak, which consists of a magnetic ‘cage’ made by strong magnetic fields which prevent ions/particles from touching the walls.
In order to produce energy, the plasma needs to be confined long enough for fusion to occur. This was first achieved with the Joint European Torus (JET) device.
Whilst JET is still operational, ITER is the next succession. Based in southern France, ITER is a collaboration of 35 nations and will be the first to produce net energy.
Decommissioning consists of difficult operations, sometimes in confined spaces. Research and innovation are needed in this challenge to ensure work dose and safety standards are upheld in the process.
For example, the use of robots and drones is becoming increasingly important, in remote working and also for characterization purposes.
Nuclear-powered diamond batteries
Researchers in the UK have looked at reusing carbon from nuclear reactor graphite blocks (previously used as a moderator) to create batteries3.
References
1 https://sckcen.be/en/SCKCEN_for_you/Energy/Gen_IV_reactors
2 ITER (2019) – What is Fusion? Accessed 08/08/19 at: <https://www.iter.org/sci/whatisfusion>
3 University of Bristol Cabot Institute – Diamond Batteries. <https://www.bristol.ac.uk/cabot/what-we-do/diamond-batteries/> Video: https://www.youtube.com/watch?time_continue=260&v=b6ME88nMnYE
Others
- Generation IV Forum (2013) – Generation IV Systems. <https://www.gen-4.org/gif/jcms/c_59461/generation-iv-systems>
- Fusion for Energy (2019) – Understanding Fusion. <https://fusionforenergy.europa.eu/understandingfusion/>
- IAEA (2019). Industrial Applications and Nuclear Cogeneration. <https://www.iaea.org/topics/non-electric-applications/industrial-applications-and-nuclear-cogeneration>
Table 1: Generation IV reactor concepts